QUANTUM OPTICAL MICROSCOPE
QUANTUM MICROSCOPES TO IMAGE THE OTHERWISE INVISIBLE
WHAT IS IT?
Quantum-light microscopes provide a unique and versatile tool to accomplish difficult sensing tasks important to the performance of the warfighter. They
exploit quantum correlations between photons to achieve images with quality beyond what is possible with conventional (classical light) techniques. They increase imaging speed, improve imaging contrast, improve signal to noise ration and minimize photodamage.
The AFOSR Biophysics Program is playing a key role in the development of quantum microscopes and in realizing their applications. The Program developed the first nonlinear quantum microscopes capable of attaining superior to any existing methods contrast of chemical, mechanical, and fluorescence imaging. The Program also showed, for the first time, that quantum microscopy can overcome photodamage, a critical constraint of precision microscopes.
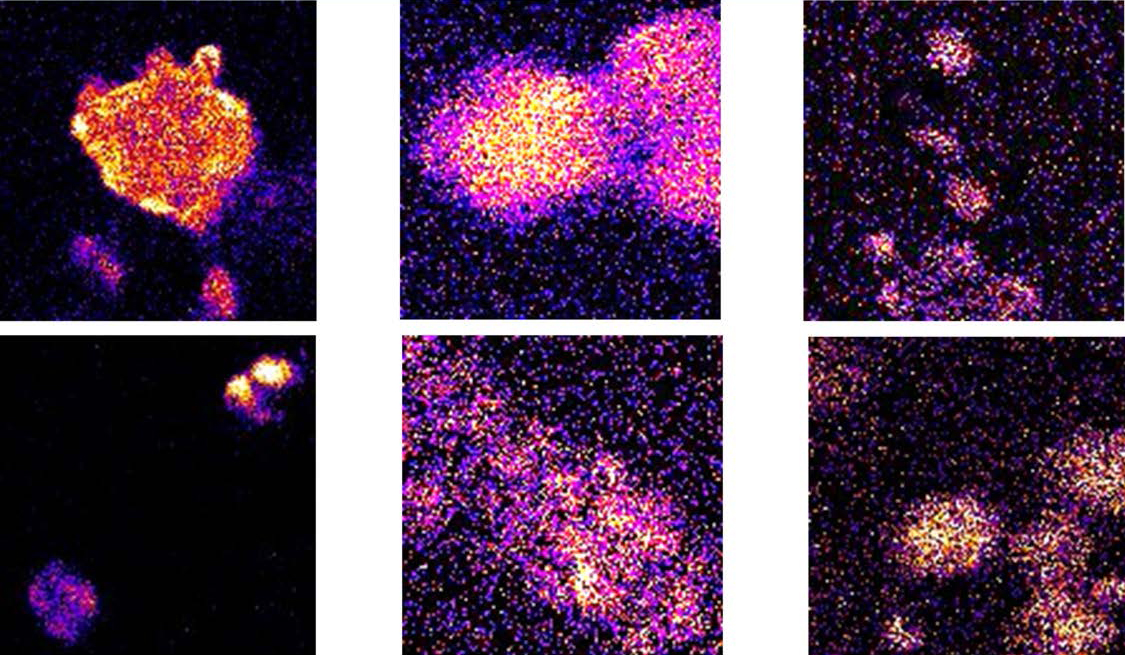
Figure 1: Entangled two-photon light microscope images of (left) MCF7 human cells stained with the dye Hoechst 34580, cells with different numbers of nuclei (middle), and a colony of human cells (right). Measurements with Classical Light of the same intensity showed no detectable images of the cells. Photo Credit: Theodore Goodson III University of Michigan, J. Phys.Chem. 2022, 13, 12, 2772-2781.
FACTS ABOUT THE QUANTUM OPTICAL MICROSCOPE
- Quantum microscopes enable contrast, signal-to-noise and speed beyond what is possible with other image techniques
- The AFOSR Biophysics Program is world leading in the development of quantum microscopes and their application to chemical, structural and fluorescent imaging
- Quantum microscopy could transform our understanding of sub-cellular life, and offer broad impact beyond bioimaging, e.g. material science,
stealth detection, and spectroscopy
HOW DOES IT WORK?
Quantum-light microscopes exploit specially-engineered light sources that contain quantum correlations between photons. These correlations can be used to both reduce the measurement noise and increase the interaction cross-section, and therefore signal strength.
Noise reduction: The measurement noise of conventional microscopy is fundamentally constrained by shot noise due to the random arrival of photons.
Quantum correlations can allow photons to arrive at the detector used in a microscope in a more regular fashion. This suppresses the measurement noise and improves the sensitivity of detection and the accuracy of such measurements.
Signal enhancement: Many microscopic imaging techniques utilize a signal generated via multi-photon interactions. They rely on the statistical chance that
the necessary photons are present in the sample at that same time. Quantum correlations can be used to remove this statistical uncertainty, greatly increasing the signal strength.
Entangled Two-Photon Microscopy: An entangled two photon microscope was constructed at the University of Michigan for the purpose of enhanced sensing, recognition of microscopic structures as well as for obtaining unique information on biological samples. Quantum microscopy provides a breakthrough over the classical two-photon microscopy by allowing orders of magnitude lower excitation intensity to be used making detections safer for biological samples and high molecular selectivity (See figure 1). Another important advantage of broadband entangled photons over the classical counterparts is a non-classical time–bandwidth property which can be used to spectrally resolve very complex spectra while at the same time maintaining ultrafast time resolution. Thus, the quantum optical microscope can be used to resolve biologically relevant features of human and non-human live cells while minimizing photodamage compared to classical light techniques. The quantum optical microscope can be used to resolve biologically relevant features of human and non-human live cells while minimizing photodamage compared to classical light techniques.
Quantum Brillouin Microscopy: The uniqueness of Brillouin microscopy is based on its ability of assess local viscoelastic properties of cells and tissues which are important to understand mechanical forces and interactions on microscopic level. In particular, the variations of local mechanical properties of brain tissues serve as a good indicator of developing health problems, such as Alzheimer’s disease of traumatic brain injury. Traditional (classical light) Brillouin spectroscopy is limited by the shot-noise limit, which diminishes the contrast and reduces the accuracy of defining local stiffness and viscosity. Quantum-enhanced Brillouin microscopy utilizing squeezed states of light was constructed at Texas A&M University and was used to improve the signal-to-noise ratio of Brillouin hyperspectral images. In particular, an image the whole brain of Drosophila melanogaster (fruit fly) was collected with higher imaging contrast.
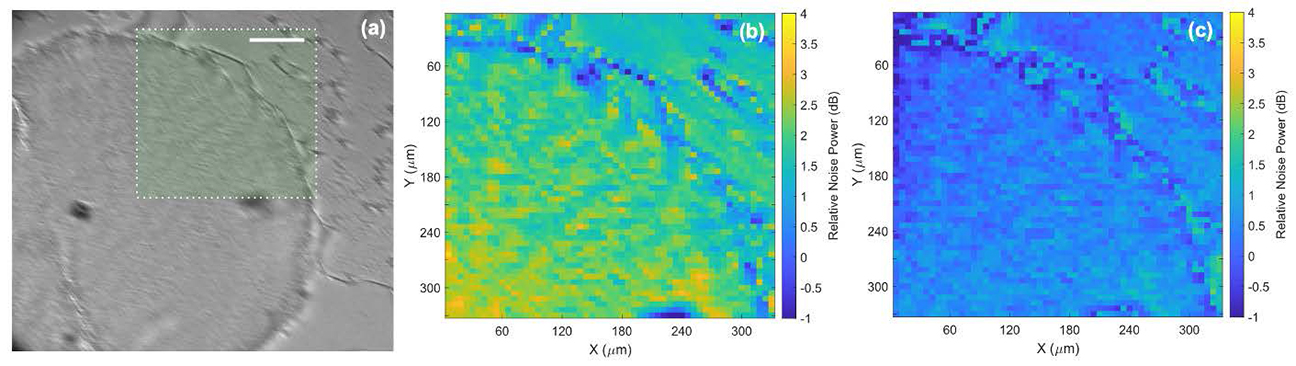
Figure 2: (a) Bright field image of a Drosophila brain (scale bar is 100 μm). (b) Quantum light Brillouin image of the selected area, and (c) classical light Brillouin image of the same area showing increasing image contrast for quantum enhanced imaging. Image Credit: Tian Li, Vsevolod Cheburkanov, Jace Willis, Girish Agarwal and Vladislav Yakovlev, Texas A&M University.
Quantum Raman Microscopy: Raman microscopy probes the vibrations of molecules in a sample to determine their chemical composition. This provides a powerful tool for characterizing materials, studying the brain, and understanding the nature of biological specimens. The AFOSR Biophysics Program developed the first quantum-enhanced Raman microscopes, employing techniques from gravitational wave detection to suppress the microscope noise floor of live-cell imaging (see Figure 3). They used these microscopes to demonstrate absolute quantum advantage in imaging for the first time – showing that quantum imaging allows the detection of biological structures that photodamage makes inaccessible to conventional microscopes.
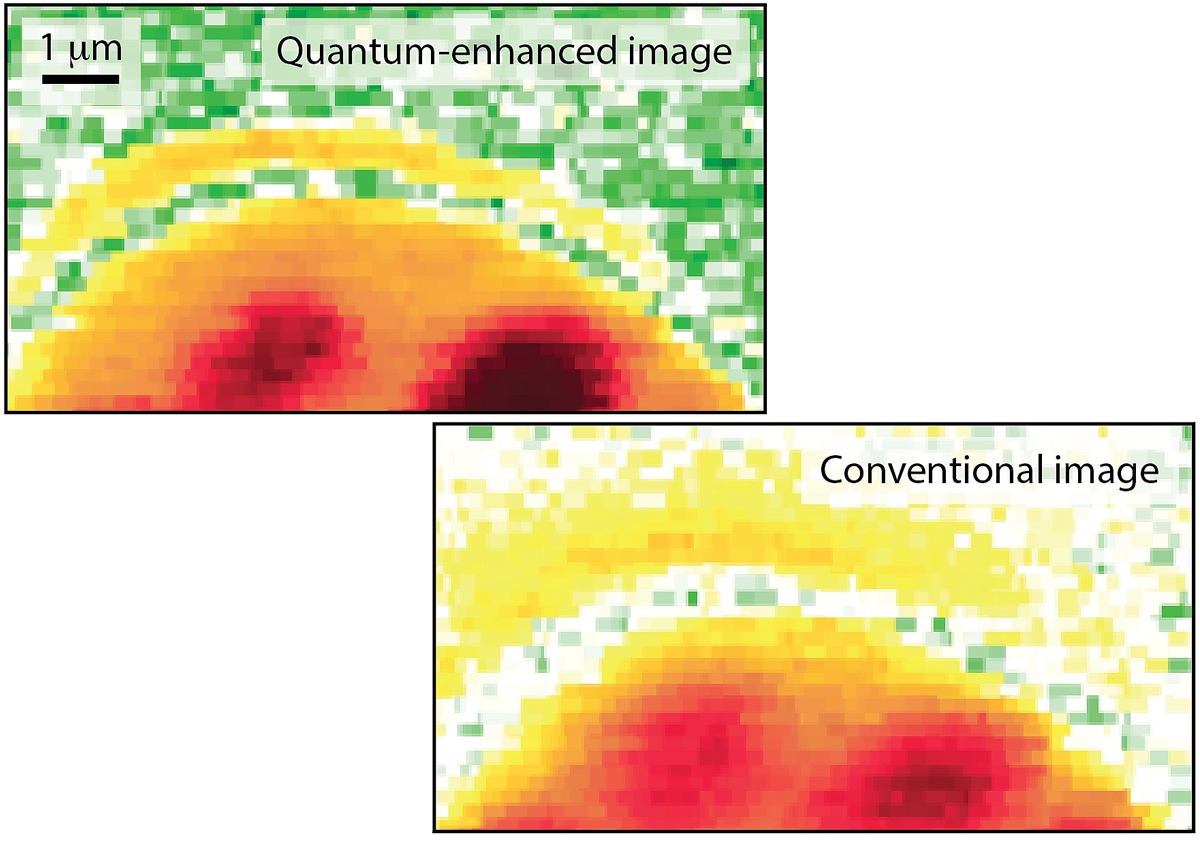
Figure 3: Comparison of conventional and quantum enhanced image of a yeast cell. From: Nature 594 201 (2021). Credit: Warwick Bowen, the University of Queensland.